When I teach sponge biology to students of invertebrate zoology, I spend a lot of time describing them as phenomenal filter feeders, and suspect that most other professors do the same. There really are no animals that come close to possessing sponges’ ability to remove very small particles from the water. Sponges have this ability despite the fact that their bodies are extraordinarily simple. I can draw pictures on the board to diagram the variety of sponge body types, but I’ve always wanted to show students how these bodies actually work.
Thing is, from the outside sponges just aren’t that interesting. Some grow into large, conspicuous tube or vase shapes, but most occur as crusts of varying thickness and color. For example:

10 September 2018
© Allison J. Gong
or this:

24 September 2018
© Allison J. Gong
Not much to write home about, is it?
But as with most things invertebrate, sponges are more complex than they appear to be at first glance. And of course their complexity can be best appreciated when you observe sponges under the microscope. That’s what I’ve been doing over the past few weeks: making wet mounts of living sponge and looking at them under the compound microscope. I’m still figuring out the best way to take photos through the scope, and trying to find the magic combination of lighting, magnification, and depth of field to obtain the clearest images.
Let’s take a step back and review some basic sponge fundamentals. Sponges are animals in the phylum Porifera. Their bodies are characterized by a lack of true tissues; in other words, a sponge’s body consists of various types of cells that do not form permanent connections. The different types of cells have different functions, but most of the cells retain the characteristic of totipotency, the ability to differentiate into another cell type as needed.
The sponge cells that do the filtering are called choanocytes. They form the lining of the sponge’s body cavity. Choanocytes consist of a cell body and a collar region of microvilli that form a ring. From the center of the ring protrudes a single flagellum, whose undulations travel from base to tip. The choanocytes are arranged so that the flagella face into the body cavity, and their collective beating draws water through the body. The flagella also capture food particles, which are phagocytosed by the cell.

© Sinauer Associates, Inc.
In its simplest tubular form, a sponge can be visualized as a miniature vase, with a single body cavity called a spongocoel (‘sponge cavity’) which is lined with choanocytes. Water enters the sponge through many microscopic pores on the outer skin of the body, is filtered by the choanocytes, and exits through a single opening called the osculum. This system works, but the efficiency of filtering is limited by the surface area of the choanocyte layer lining the spongocoel, and very few sponges have this body type.
Now if you imagine making invaginations into the choanocyte layer and continue the choanocytes into the channels you create, you could increase the filtering surface area of a sponge without having to increase its overall body size. Continue this maneuver to its logical end and you’d end up with something that resembles a cluster of grapes. The skin of the grapes would represent the layer of choanocytes, all oriented so that their flagella face the hollow interior of the grape, which would correspond to what we call a choanocyte chamber. This type of body plan has a vastly expanded surface area to volume ratio compared to the tubular form, and these sponges achieve the largest sizes. Incidentally, natural selection has used this exact same strategy to maximize the respiratory exchange surface area of your lungs: gas exchange occurs in the alveoli, which are tiny thin-walled sacs where oxygen diffuses into and carbon dioxide diffuses out of capillaries. The total respiratory surface area of your lungs is about 70 m2—i.e., roughly equivalent to one side of a standard tennis court, without the doubles lanes—all tucked neatly into the volume of your thoracic cavity.
The canals leading into and out of each choanocyte chamber are smaller than the chamber itself, and this arrangement takes advantage of some fundamental fluid dynamics: a given volume of water flows faster through a tube with a narrow diameter and slower through a tube with a wider diameter. Water travels relatively fast through the narrow canals on either end of a choanocyte chamber and slows down significantly within the chamber proper. This gives the choanocytes time to capture all of the food particles in the water stream, and speeds the water to the outside of the body once it has been filtered.
Now we can get back to the animals themselves. Their external appearance may not look like much, but sponges are very interesting when viewed under a microscope. I’ve been taking samples and squashing them under coverslips for a close look.
Here’s a view under darkfield lighting:
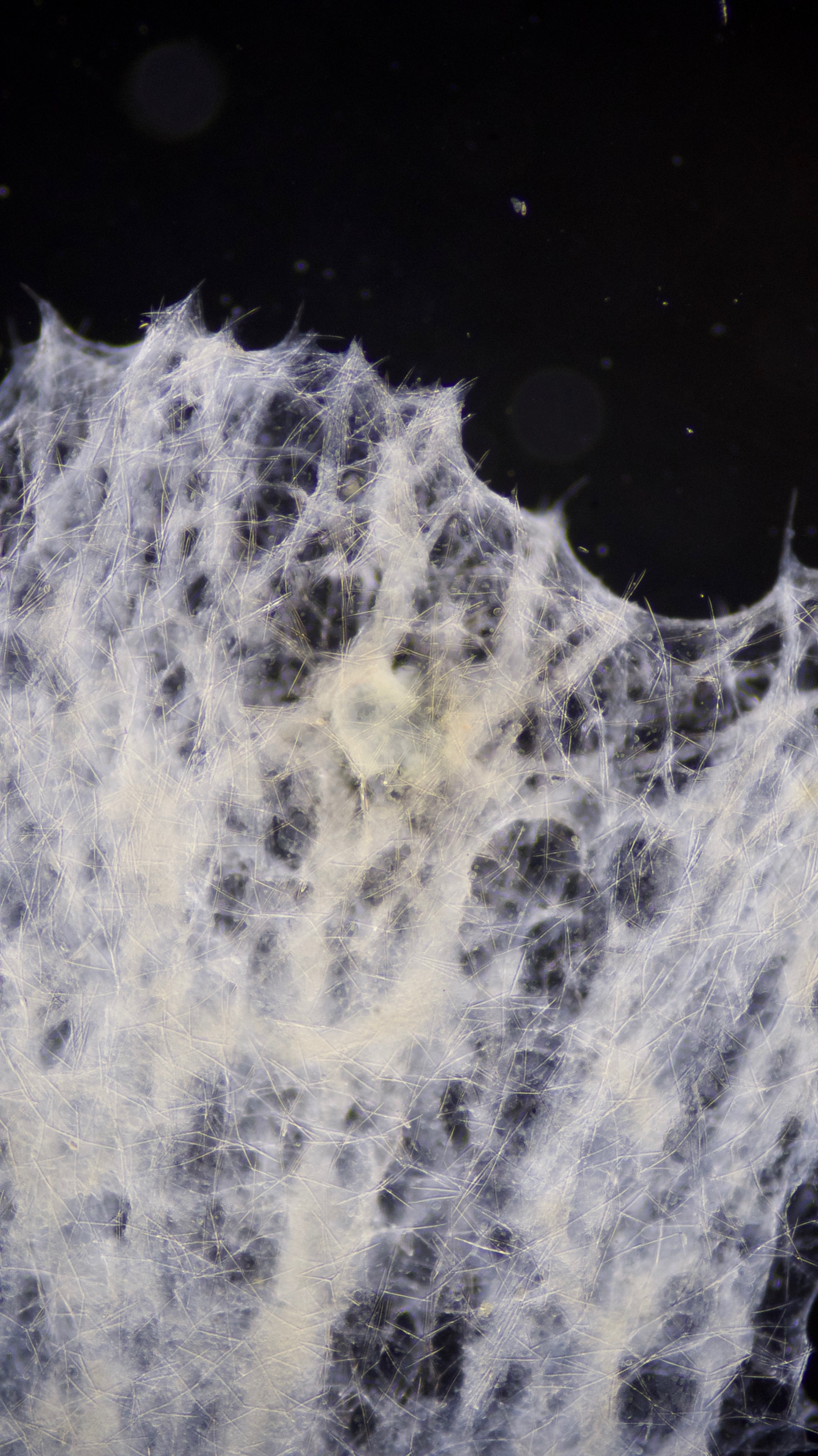
8 September 2018
© Allison J. Gong
The clear-ish objects that look like the back roads of a map are spicules. They provide a bit of skeletal support for the sponge’s body and help to deter predators–who would want to bite a mouthful of glass splinters?
When I switched to higher magnification and phase-contrast lighting I could see hollow spherical structures that vaguely resembled blackberries. I felt a thrill of excitement to realize that these were probably choanocyte chambers, and I was looking at the choanocytes themselves!

8 September 2018
© Allison J. Gong
Here’s another view at the same magnification, which shows more clearly the cells of the chamber:
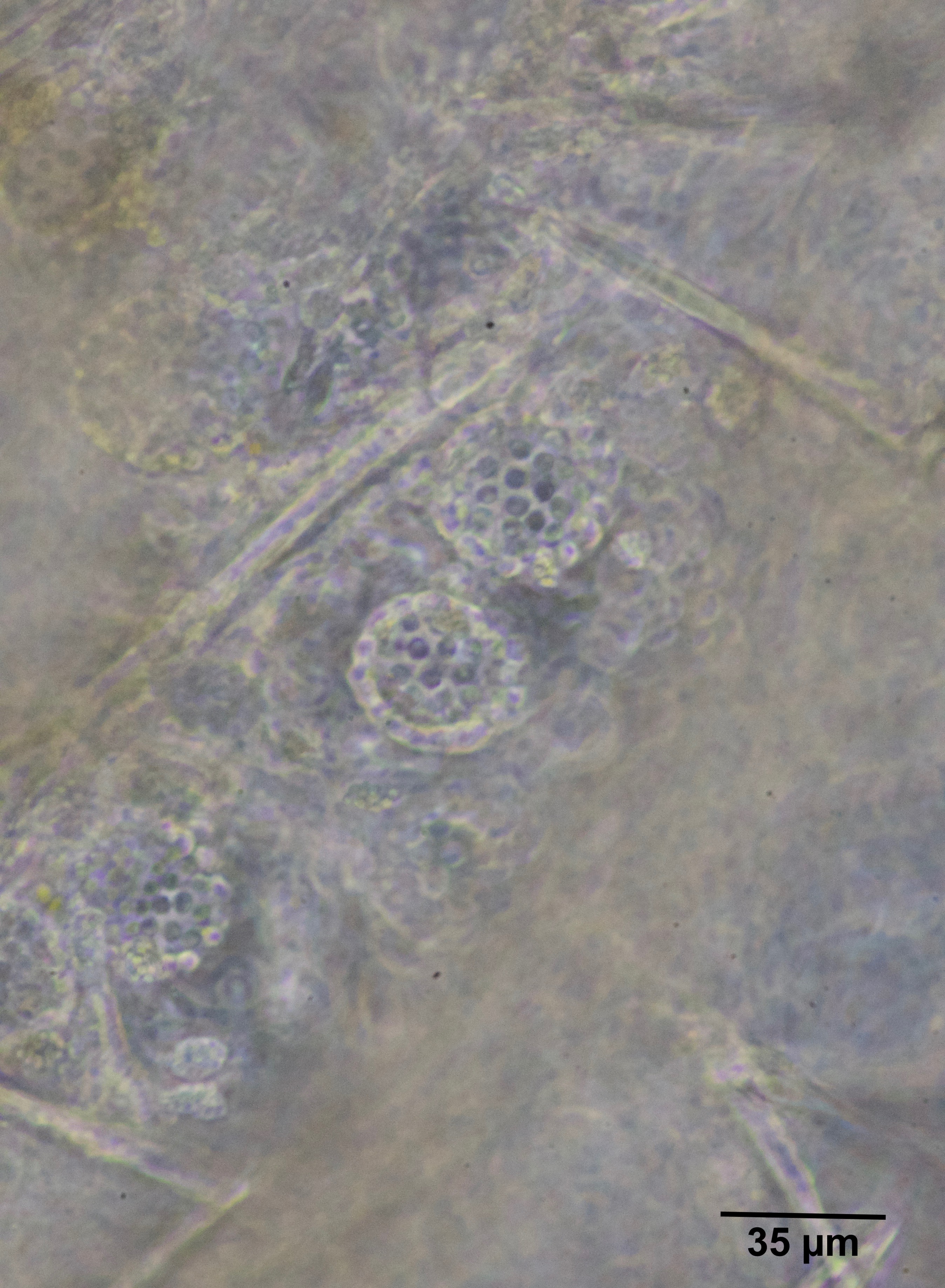
8 September 2018
© Allison J. Gong
The chambers themselves closely resemble the blastula stage of early animal embryology. Like a blastula, a choanocyte chamber is a hollow ball of cells; unlike a blastula, which has a ciliated outer surface, a choanocyte chamber consists of flagellated cells with the flagella oriented towards the inner hollow space. At a bit less than 40 µm in diameter, the chambers are about half the size of my sea urchin blastulae.
Remember how I said that the structure of the choanocyte chambers is similar to that of our alveoli? You may not be able to visualize the alveoli in your lungs, but this photo shows how the chambers resemble a cluster of grapes.

22 October 2018
© Allison J. Gong
Because it’s impossible to see the three-dimensional structure of the chambers from the single plane of focus you get with a photograph, I shot some video while focusing up and down through the sample on the slide.
They really do look like grapes, don’t they?