It has taken me months to gather all the photos and videos I needed for this post. I could blame it on the stress of teaching online for the first time, the COVID-19 pandemic itself, or residual malaise from the dumpster fire that was 2020. But really, it’s the animal’s fault.
In this case the animal is the orange cup coral, Balanophyllia elegans. I’ve written about this beast before, and lately I’ve been paying more attention to the corals that we have in the lab. In many ways it is the typical anthozoan—its life cycle consists of only a polyp stage (i.e., no medusa), it is benthic, and its body is vaguely anemone-like. Like the reef-building corals of the tropics, Balanophyllia is a scleractinian coral. This means that it secretes a calcareous base, or exoskeleton, upon which sits the living tissue of the polyp. But unlike the reef-building corals of the tropics, Balanophyllia is solitary, which means that it does not clone or form colonies. Nor does it contain photosynthetic zooxanthellae in its cells, as the reef-builders do. This means that Balanophyllia is a strict carnivore: unable to rely on photosynthetic symbionts to do the hard work of fixing carbon, Balanophyllia has to catch its own prey.
Balanophyllia has separate sexes and reproduces sexually. Males release sperm that are ingested by the female, and she then broods larvae for several months. What is strange about this is that, when you consider the anatomy of Balanophyllia (or any cnidarian polyp, for that matter) the question that comes to mind is, “Where are the larvae brooded?” So let’s take a look at the basic cnidarian body plan.
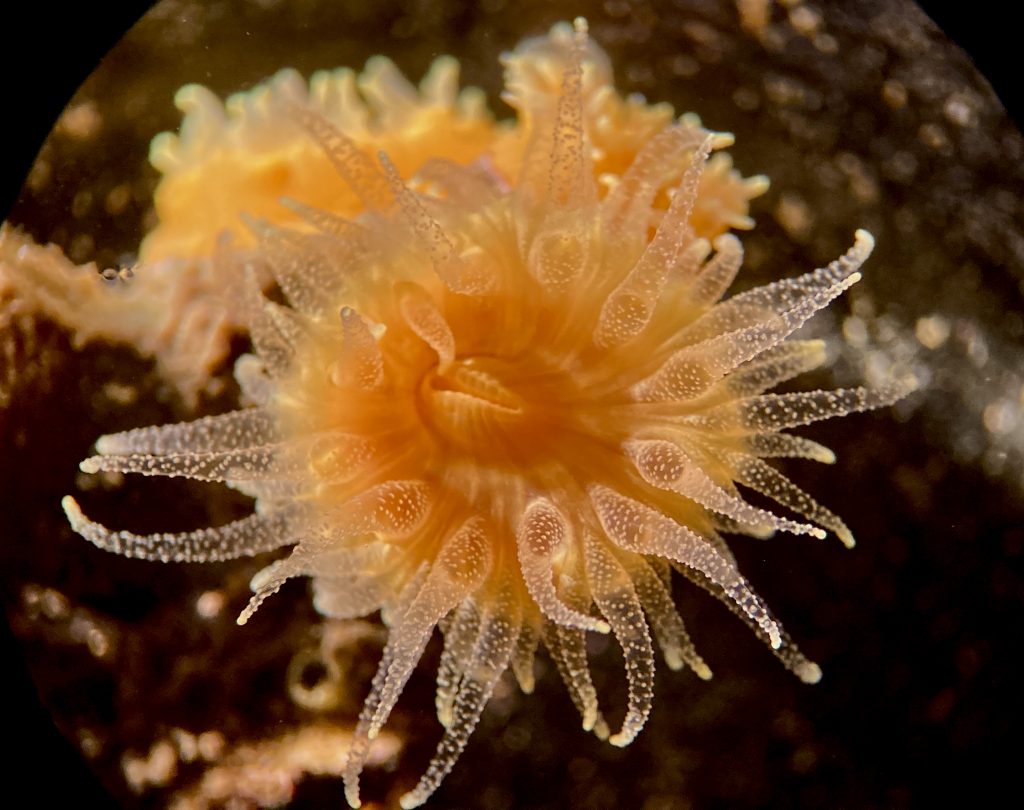
© Allison J. Gong
The professor who taught my undergraduate course in invertebrate zoology described a cnidarian’s body as a baggie inside a baggie, with a layer of jelly between the two baggies. The inner and outer baggies represent the tissue layers that developmental biologists refer to as endoderm and ectoderm, respectively. The jelly between the two tissue layers is called mesoglea. For the sake of this discussion, it’s the endoderm that interests us. In a cnidarian, the endoderm is also called gastrodermis, because it is, literally, the skin of the digestive system. The cnidarian gut is a cavity that opens to the outside via a single opening. We could call that opening either a mouth or an anus, since both food and wastes pass through it, but for politeness’ sake we call it a mouth. The gut itself does double duty as both the digestive system and the circulatory system, so its formal name is gastrovascular cavity (GVC).
It’s easy to imagine the GVC as being essentially a tubular vase, but it’s more complex than that. The GVC extends into each of the tentacles, so the tentacles are actually hollow. The main cavity of the GVC, in the stalk of the polyp, is partitioned by thin layers of endoderm. The partitions are called septa, and serve to increase the surface area of the endoderm for digestion. Think of a large office building, divided into small cubicles by movable partial walls—there’s much more total wall space for hanging things such as calendars than there would be in the big room with only four walls. In anthozoans, gonad tissue is associated with the septa.
Now back to how Balanophyllia broods its larvae. As we’ve seen before, Balanophyllia‘s planula larva is an orange-reddish wormlike blob about 2 mm long, which is ciliated all over. It doesn’t feed, but survives on energy reserves supplied by the mother in the egg; it may also be able to take up dissolved organic material directly from the seawater. After brooding larvae for some period of time in their GVC, Balanophyllia females release larvae. Or rather, the larvae ooze out of their mother’s mouth and crawl around to settle and metamorphose nearby.
The planulation season, when larvae show up amongst the corals we keep in the lab, begins in the fall and runs through winter into the spring. Sometimes the larvae metamorphose right away—one day there are no larvae and the next day there are baby corals. Other larvae squirm around for days or weeks, not getting any smaller but not metamorphosing, either. This past season (Fall 2020-Spring 2021) we saw both extremes: planulae that settled and metamorphosed right away, and others that I collected weeks ago and are still worming around.
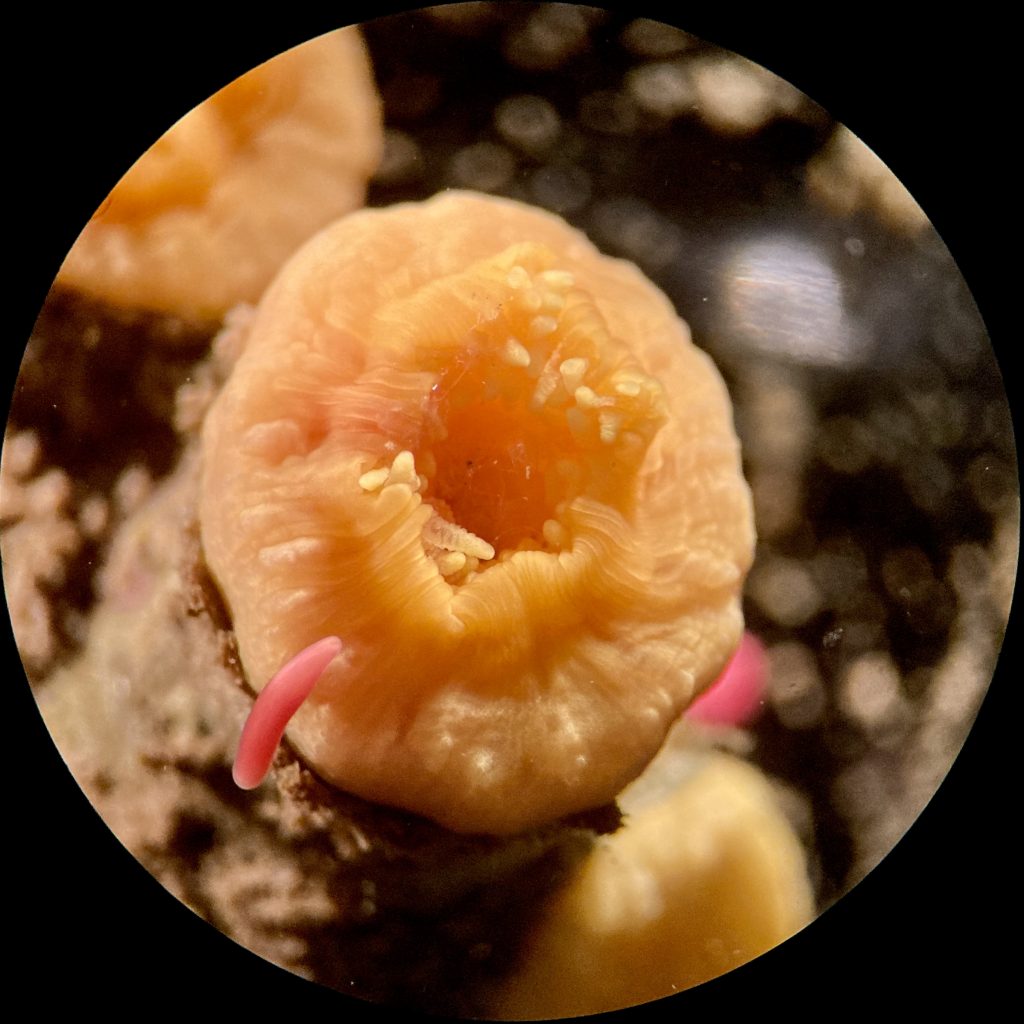
2020-12-12
© Allison J. Gong
I peered into a bunch of corals, hoping to see what the larvae are doing inside the GVC, with no luck. Then I decided to try some time-lapse video under the dissecting scope, and had a bit of success with that. In the video below you’ll see about two hours of action compressed into about 1 minute. Watch for a small red ball squirming around inside the GVC. And remember that the GVC extends into the tentacles, so the larva can wiggle its way in there, too.
All of which makes me wonder if the pathway from mother’s GVC to the scary world outside travels in only one direction, or if the larvae can retreat back into the safety of the mom’s digestive system. How strange is it, that the safer location might be inside the mother’s gut!
Eventually, whether it be a matter of hours or weeks, the planula larva settles (i.e., sticks to a surface and stops crawling) and metamorphoses (i.e., makes the anatomical and physiological changes into the juvenile body). There doesn’t seem to be a clear connection between surface characteristics and whether or not larvae will settle there. You might think that they’d choose to settle nearby or maybe actually on the parent, but that’s not always the case. At some point, however, the larva will have to either settle and metamorphose, or die. When they metamorphose into juveniles, they reveal other aspects of their nature as scleractinian corals.
From what I’ve seen, the larva begins the settlement process by sticking to a chosen spot and becoming a more circular (i.e., less elongated) blob. On the other hand, I’ve seen perfectly round red blobs that look for all the world as though they might be pushing out tentacles, change their minds and go vermiform again. But once they stick permanently and begin to metamorphose by forming the polyp’s body, they have to continue.
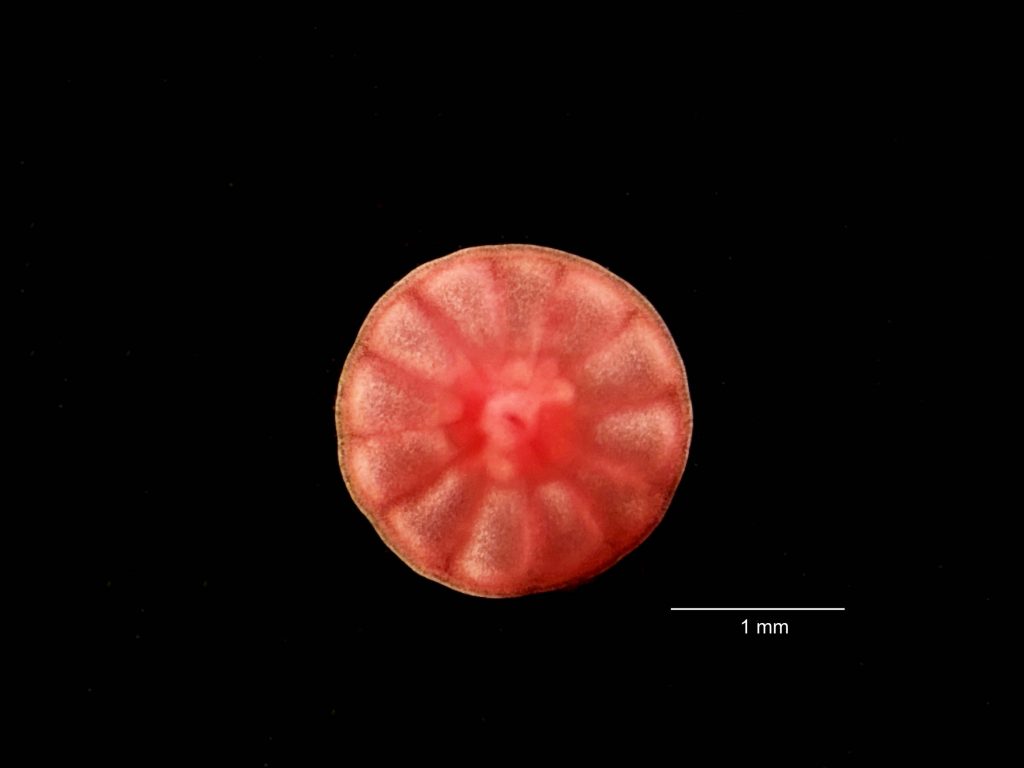
2021-01-29
© Allison J. Gong
The juvenile in the photo above has not yet pushed out any tentacles, but it does show signs of its scleractinian ancestry. The Scleractinia (stony corals) and Actiniaria (sea anemones) are both members of a group called the Hexacorallia. The Hexacorallia and Octocorallia are in turn grouped together as members of the class Anthozoa. As you might infer from the name ‘Hexacorallia’, animals in this group of a body symmetry based on the number six. And you can see in the photo above that the juvenile coral’s body is divided into 12 wedges. When the juvenile begins growing tentacles and a more polyp-like body, the hexamerous symmetry becomes even more evident:
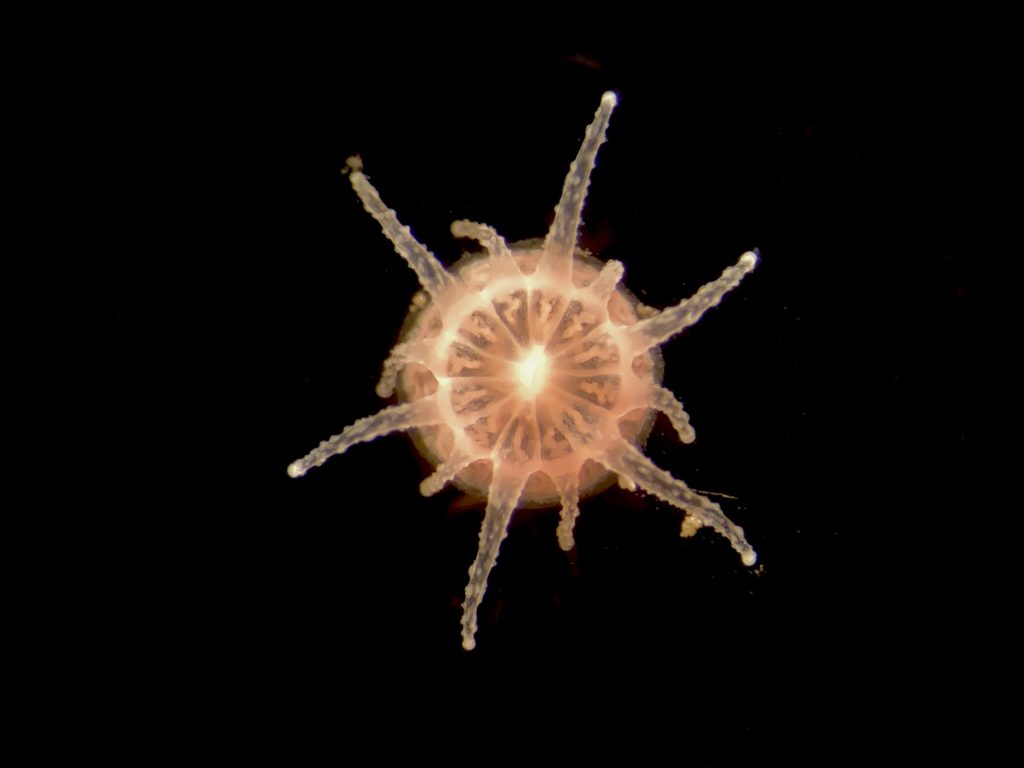
2021-02-22
© Allison J. Gong
The young coral begins by forming six primary tentacles, which establishes the visible hexamerous symmetry. Then it grows the six shorter secondary tentacles. The bumps on the tentacles are cnidocyte batteries, clusters of stinging cells, indicating that the animal can already capture and kill prey. Good thing, too, because if it hasn’t already then it soon will deplete the energy stores its mother partitioned into its egg.
By this stage in its development the coral will remain where it is for the rest of its life. As it grows it will deposit CaCO3 and grow taller, but the living tissue will always be restricted to a layer sitting on top of the calcareous base. Once the base grows more than a few millimeters tall, there is no living tissue in contact with the surface. Thus there is no mechanism for the animal to move to another location, or even to re-attach itself if it gets broken off its surface. There are many animals that are likewise unable to move once their larvae have attached and metamorphosed. The decision of where to put down roots becomes quite stark for them, as a bad one can result in a very short life indeed. The selective pressures that enforce a good decision are quite clear, and are important factors in the distribution patterns we observe in the intertidal.