When it comes to the natural world, I have always found myself drawn to things that are unfamiliar and strange. I think that’s why I gravitated towards the marine invertebrates: they are the animals most unlike us in just about every way imaginable. Even so, some of them have bodies at least that are recognizable as being both: (1) alive; and (2) animal-ish. Think, for example, of a lobster and a snail. Each has a head and the familiar bilateral symmetry that we have. Obviously they are animals, right? I, of course, am most fascinated not by these easy-to-understand (not really, but you know what I mean) animals, but to the cnidarians and the echinoderms. And for different reasons. The cnidarians astound me because they combine morphological simplicity with life cycle complexities that boggle the mind. I hope to write about that some day. Today’s post is about my other favorite phylum, the Echinodermata.
For years now I’ve been spawning sea urchins, to study their larval development and demonstrate to students how this type of work is done. I have a pretty good idea of what to expect in urchin larvae and can claim a decent track record of raising them through metamorphosis successfully. Urchins are easy. To contrast, I have much less experience working with sea stars. I have found that some species are easy to work with, while others are much more problematic. Bat stars (Patiria miniata), for instance, are easy to spawn and raise through larval development into post-larval life. Ochre stars (Pisaster ochraceus), on the other hand, go through larval development beautifully, but then all die as juveniles because nobody has figured out what to feed them. I’ve already chronicled my and Scott’s attempts in 2015 to raise juvenile ochre stars in a series of posts starting here.
Sea urchins and sea stars have long been model organisms for the study of embryonic development in animals, for a few reasons. First, many species of both kinds of animals are broadcast spawners, which in nature would simply throw their gametes out into the water. This means that development occurs outside the mother’s body, so biologists can raise the larvae in the lab and observe what happens. Second, spawning can be induced by subjecting the parents to nonlethal chemical or environment stresses. Third, the larvae themselves are often quite happy to grow in jars and eat what we feed them. Fourth, the larvae of the planktotrophic species are often beautifully transparent, allowing the observer to see details of internal anatomy. Lucky me, I’ve been able to do this several times. And it never gets old.
All that said, there are differences between urchins and stars that force the biologist to treat them differently if we want them to spawn. For the species I work with, spawning occurs after I inject a certain magic juice into the animals’ central body cavity–urchins get a simple salt solution (KCl, or potassium chloride) and stars get a more complex molecule (1-MA, or 1-methyladenine). The fact that you can’t use the same magic juice for urchins and stars reflects a fundamental difference in gametogenesis and spawning in these groups of animals.
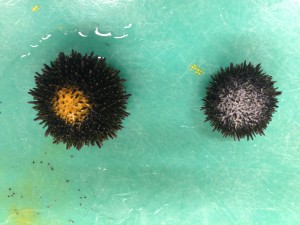
20 January 2015
© Allison J. Gong
Sea urchins will spawn only if they have fully developed gametes. In other words, gametogenesis must be complete before gametes can be released to the outside. You can inject as much KCl into a sea urchin as you want, but if it’s the wrong time of year or the urchin doesn’t have mature gonads (due to poor food conditions, perhaps), it won’t spawn. I’ve never investigated the mechanism by which KCl induces spawning in ripe urchins, but here’s what I think happens.
When students dissect animals in my invertebrate zoology class, we use magnesium chloride (MgCl2) to narcotize the animals first. A 7.5% solution of this simple salt is remarkably effective at putting many animals gently to sleep, especially molluscs and echinoderms. Placing the animals in a bowl of MgCl2 and seawater causes them to relax and gradually become unresponsive. A longer bath in the MgCl2 puts them to sleep for good.
Given the relaxation effects of MgCl2 on urchins, I suspect that injecting a solution of KCl into the body cavity relaxes the sphincter muscles surrounding the gonopores. This relaxation opens the gonopore, and if the gonads are ripe the mature gametes are released to the outside. As I said above, I don’t know for certain if this is how it works, but the hypothesis makes sense to me. It also explains why that I can shoot up a dozen urchins and get none of them to spawn: the KCl might be doing what it normally does (i.e., opening the gonopores) but if the gonads aren’t ripe there are no gametes to be released.
For completely different reasons, injecting a star with KCl does absolutely nothing at all except probably make the animal a bit uncomfortable. The KCl may very well open gonopores as it does in urchins, but a star will never have mature gametes, especially eggs, to release in response to this muscle relaxant. This is because at least in female stars, meiosis (the process that produces haploid gametes) isn’t complete until the eggs have been spawned to the outside. What, then, is the magic juice used to induce spawning in stars, and what exactly does it do?
The magic juice is 1-methyladenine, a molecule related to the nucleobase adenine, most commonly known as one of the four bases that make up DNA. The nomenclature indicates that the difference between the two molecules is the addition of a methyl group (–CH3) to the #1 position on an adenine molecule:
Chemistry aside, what I’m interested in is the action of 1-MA on the eggs of sea stars. Meiosis, the process that produces gametes, has two divisions called Meiosis I and II. Meiosis I starts with a diploid cell (i.e., containing two sets of chromosomes) and produces two diploid daughter cells; these daughter cells may not be genetically identical to each other because of recombination events such as crossing over. It isn’t until Meiosis II, the so-called reduction division, that the ploidy number is halved, so each daughter cell is now haploid (i.e., containing a single set of chromosomes) and can take part in a fertilization event. In a nutshell, the end products of meiosis are haploid cells, all of which ultimately result from a single diploid parent cell.
In female sea urchins, the entire meiotic process is completed before the eggs are spawned, which is why the relaxation effects KCl can induce spawning.
In females of many other animal species, meiosis is arrested for some period of time after the Meiosis I division. For example, this happens in humans: baby girls are born with all of the eggs they will ever produce, maintained in a state of suspended animation after Meiosis I. It isn’t until puberty that eggs begin to complete meiosis, one egg becoming mature and being ovulated approximately monthly for the rest of the woman’s reproductive life. Sea stars are sort of like this, with the notable exception that a female star will ripen and produce thousands of eggs in any spawning event rather than doling them out one at a time.
One of the really cool things about working with sea star embryology is that I get to see the completion of meiosis after the eggs have been spawned. I know that the gonads have to reach a certain level of ripeness before 1-MA will induce spawning. Reviewing my notes from a course I took in comparative invertebrate embryology when I was in graduate school, I came across the mention of ‘polar bodies,’ tiny blobs that I remember seeing in just-fertilized sea star eggs but which I have never seen in sea urchin embryos. Then I needed to remind myself what polar bodies are all about.
Remember how there are two cell divisions in meiosis? Well, despite what’s shown in the diagram above, each of the divisions is asymmetrical. In other words, each division of meiosis produces one big cell and one tiny cell. The tiny cells are the polar bodies. They are too small to either divide or be fertilized, and generally die on their own. Here’s a chronology of what happens. First, a cell divides, producing a large cell and a tiny polar body:
I’ve x’d out the polar body in red because it cannot divide or be fertilized and will soon die. Then the large cell divides to produce the final egg and a second polar body:
It turns out that in sea stars things get even more complicated. 1-MA acts as a maturation-inducing substance in these animals, effectively jump-starting the eggs that have been sitting around in an arrested state after undergoing Meiosis I. This initiates the continued maturation of the eggs to the stage when they can be spawned. Even now, though, meiosis doesn’t complete until an egg has been fertilized, at which point the second polar body is produced. The production of that second polar body is the signal that Meiosis II has occurred, and the now-fertilized egg can begin its embryonic development.
Here’s a freshly fertilized egg of Pisaster ochraceus, with the two polar bodies smushed into the narrow perivitelline space between the surface of the zygote and the fertilization envelope:
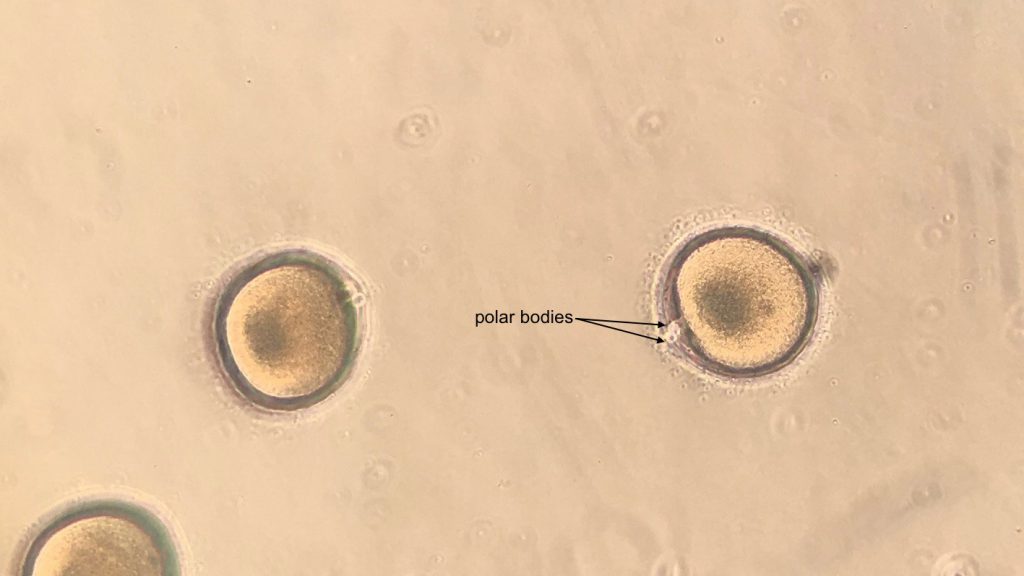
25 April 2017
© Allison J. Gong
Sea urchins, remember, do not have polar bodies when I spawn them. That’s because meiosis is complete by the time the eggs can be spawned, so the polar bodies have already died or been resorbed by the final mature egg. The photo of the P. ochraceus zygotes was taken within a few minutes of fertilization. Let’s contrast that with a photo of a brand new urchin zygote:
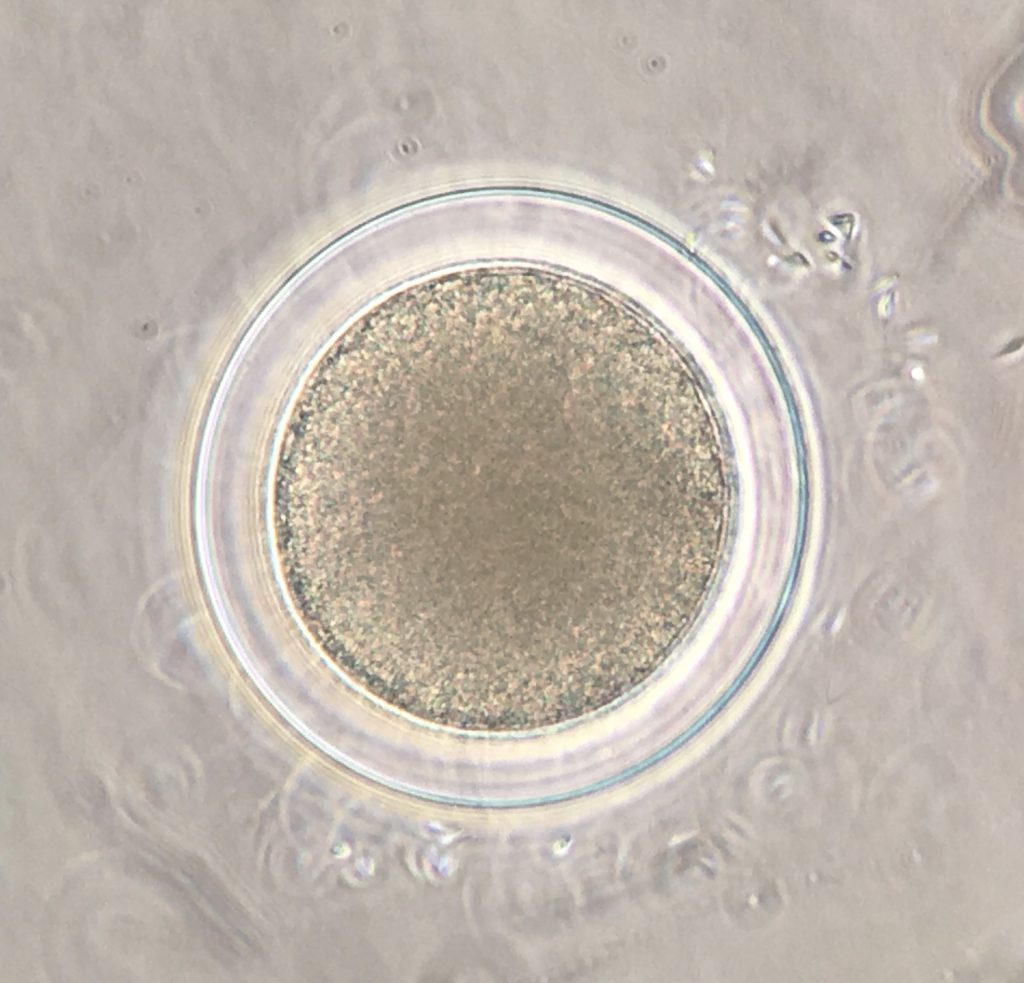
30 December 2016
© Allison J. Gong
See? No polar bodies!
All of this is to explain why we can’t use the same magic juice to spawn both urchins and stars. Kinda cool when the madness in our method has a biological context, isn’t it?